A two-pronged approach offers new insights into HIV
Among the researchers contributing to the revolution is Dr. Scott Blanchard, associate director of the Tri-Institutional Chemical Biology Program, whose lab utilizes such single-molecule imaging techniques to gain further insight into complex, ever-changing biological processes. "It's much easier to simplify your understanding of a system when you watch one molecule at a time, rather than billions at a time," explains Dr. Blanchard, an associate professor of physiology and biophysics. "If you're looking at many of them, it's like trying to listen to a conversation with a billion people talking at once."It's been dubbed the "super-resolution revolution": the development of biomedical imaging tools strong enough to see individual molecules — units so miniscule, they're smaller than the wavelength of light. Such technologies are transforming the way scientists look at living cells, examining the tiniest molecular details in the search for new disease-fighting treatments.
In two parallel studies published last fall in Science and Nature, Dr. Blanchard and his colleagues revealed how they modified this super-resolution technology to track — for the first time — the precise movements of proteins on the surface of the human immunodeficiency virus (HIV). They were also able to use a method known as X-ray crystallography to determine the 3D structure of one of those proteins. Together, these discoveries could help researchers find a way to prevent HIV infection, stemming an epidemic that the WHO estimated has affected 78 million people worldwide.
For the Science study, Dr. Blanchard's group adapted single-molecule fluorescence resonance energy transfer (smFRET) imaging — an approach that uses fluorescence to measure distance on an atomic scale — to analyze the behavior of HIV. The team designed special long-lasting fluorescent molecules, or fluorophores, which were inserted into proteins on the virus's outer coating, known as envelope proteins. These glowing beacons, in conjunction with the imaging system, allowed them to visualize how the molecules moved over time, as the virus proteins changed shape.
This was important because the envelope consists of multiple proteins, referred to as trimers, that blossom like a flower when HIV comes in contact with immune cells carrying CD4 receptors, which the virus uses to enter the cell. There are 10 to 20 trimers on the surface of each HIV particle, which quickly mutate, making it hard for the body to combat the disease — and challenging researchers working to develop a vaccine. "One of the problems with HIV," says Dr. Blanchard, "is that the rate of infection and mutation is faster than your immune system can keep up."
Scientists long thought that the trimers remain passive when CD4 isn't present. But Dr. Blanchard's group found that the envelope proteins constantly shape shift, or "dance" — even without CD4. Even more striking, he and his colleagues showed that the introduction of certain antibodies stopped the envelope from opening, thereby lowering the infection rate. Tests with a small-molecule drug under development by Bristol-Meyers Squibb to prevent HIV produced similar results. "Now we know the rule — or at least, we have a hint of the rule — on how to inhibit the virus," Dr. Blanchard says. In other words, these findings could aid pharmaceutical companies in screening for more effective medications in the future, by providing a better "road map" of the virus. "If you're driving cross-country in a car but you don't have a compass or any understanding of which way is west or east, it's really difficult," he says. "We think that our approach is going to make the search for therapeutic interventions more directed."
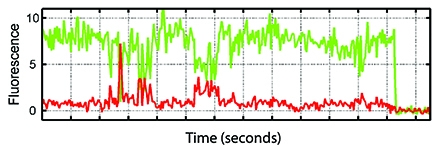
A fluoresence recording visualizing the movements of an envelope trimer complex.
In the other study, outlined in Nature, researchers turned to X-ray crystallography in an attempt to take a high-resolution picture of an HIV protein, with the goal of obtaining additional data about the virus's biological makeup. However, the procedure couldn't work if the molecule remained dynamic; as Dr. Blanchard puts it, "The nemesis of crystallography is movement." To freeze that molecule's actions, the team employed the same antibodies that were used in the Science study to keep the envelope protein closed.
To Dr. Harel Weinstein — the Maxwell M. Upson Professor of Physiology, chair of the Department of Physiology and Biophysics, and director of the Prince Alwaleed Bin Talal Bin Abdulaziz Alsaud Institute for Computational Biomedicine — the combination of these two technologies is what makes Dr. Blanchard's endeavors extraordinary. Not only do scientists now have concrete information about the structure of HIV molecules, he notes, they know exactly how they change when different proteins in the virus interact. And that knowledge could have benefits beyond HIV, pointing the way toward improved therapies for cancer and other illnesses. "The idea," says Dr. Weinstein, "is that it's only at this detailed level that we can hope to get cures."
— Heather Salerno